The capability to follow electron motion in solids is a necessary condition to
explore the ultimate speed limits of optical charge manipulation and signal processing
in opto-electronic devices. In solids, very fundamental processes like energy, charge and momentum transfer happen within the first femtoseconds after excitation: these physical mechanisms are of paramount importance, since they dominate the material response at short times after and during interaction with light, and they dictate the evolution of electron, spin and structural dynamics on longer time scales.
Since these dynamics unfold on attosecond (1 as = 10-18 s) or few femtosecond (1 fs = 10-15 s) time scales, they cannot be addressed by conventional time-resolved experiments. The main topics of this research field are focused on the development of novel attosecond beamlines and their application to the study of electron motion in solids:
Petahertz charge carrier motion in solids
Is there a fundamental limit to the speed with which information can be created and manipulated? Which is the best strategy to overcome the speed of actual electronic devices, ranging in the Terahertz (1012 Hz) regime? To answer such extremely interesting questions, several experiments have been designed in the last years to exploit ultrashort light pulses for the creation and manipulation of microscopic currents in solid-state materials.
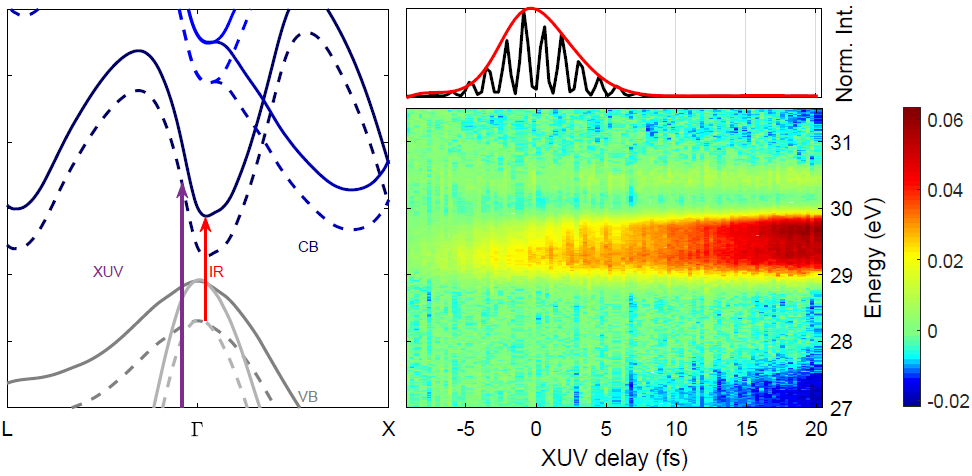
The extremely intense electric field carried by few-optical-cycle laser pulses allows for strong, direct interaction with the charge-carriers inside the material, which can be excited and controlled on the time scale of the electric field oscillations which, with visible or near-infrared pulses, approaches the Petahertz (1015 Hz) regime. The generation of such ultrafast microscopic currents has been demonstrated in insulators [2] and semiconductors [3] using attosecond spectroscopy in the extreme ultraviolet (XUV) range. The novel experimental setup developed for AuDACE will give the possibility to study, with attosecond precision, charge carrier motion in more complex materials (e.g. heterostructures, low-dimensional), key enablers for the new generation of optoelectronic devices.
Sub-fs exciton dynamics
Excitons, the quasi-particles created by Coulomb interaction between an excited electron and a hole, dominate the optical response of a wide range of materials, from organic compounds to semiconductors, making them one of the hottest topic in condensed matter physics. As attosecond spectroscopy of exciton dynamics is a relatively new field, at first we will concentrate on short-living core excitons [4] to establish the investigation techniques and study fundamental physics phenomena in conventional materials. Later we will move to advanced materials like 2D semiconductors whose electro-optical response is dominated by excitonic features. AuDACE will focus on the study of the early stage of exciton formation which takes place in the first femtoseconds during the interaction with an optical pump. This will allow to unveil founding aspects of Coulomb interaction, dynamical screening and electron correlation.
Generation of attosecond XUV pulses with arbitrary polarization
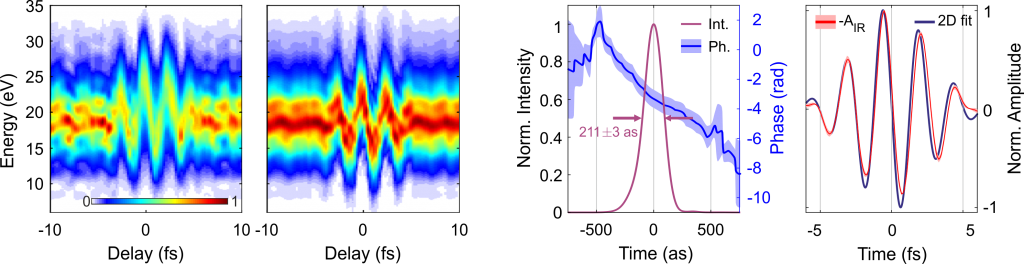
Attosecond XUV spectroscopy constitutes a powerful experimental technique to study electron dynamics in condensed matter. On one side, the temporal resolution allows to follow these phenomena in real time. On the other side, XUV photons permit to probe core electronic levels, hence providing element-specific information. Another degree of freedom which can be considered is the polarization of the probe pulse, a key ingredient to study any type of chiral medium, as in the case of magnetic materials. One of the goals of AuDACE is to achieve the full control of the polarization state of isolated attosecond XUV pulses by acting directly on their generation. During the project we will investigate new generation schemes to optimized the generated flux and degree of polarization.
Sub-fs electron and spin dynamics in ferromagnetic materials
A strong laser field can interact with a ferromagnetic material by changing its degree of magnetization on time scales on the order of tens-hundreds of femtoseconds. The effect is called laser demagnetization and is a consequence of the energy transferred from the laser to the system, which leads to an incoherent loss of magnetic interactions in the spin system. However, only in the last years a theoretical study [5] has proposed a mechanism (called Optically-Induced Spin TRansfer) to manipulate the magnetization of a ferromagnet through a direct interaction with a laser field, i.e. a coherent mechanism. AuDACE aims at employing its cutting-edge experimental setup to study electron and spin dynamics in technologically relevant magnetic materials, like low-dimensional ferromagnet. The results will open the way towards the implementation of these materials as building blocks of the new era of optoelectronic devices.
References
- Lucarelli et al., Rev. Sci. Instrum. 91, 053002 (2020)
- Lucchini et al., Science, 353, 6302, 916-919 (2016)
- Schlaepfer et al., Nature Physics, 14, 560-564 (2018)
- Lucchini et al., arXiv:2006.16008 (2020)
- Dewhurst et al., Nanoletters, 18, 3 (2018)
Collaborations
We collaborate with a number of international research groups, active in the field of attosecond technology.
- Luca Poletto (IFN-CNR, Padova, Italy)
- Angel Rubio (Max Planck Institute for the Structure and Dynamics of Matter, Hamburg, Germany)
- Shun-Suke A. Sato (Center for Computational Sciences, University of Tsukuba, Japan)
- Xiaoyang Zhu (Columbia University, New York, USA)
Further information
This research is part of the ERC funded project AuDACE: http://www.audaceproject.it/